Part 3
Insulin resistance is often thought of as a metabolic condition affecting the liver, muscles, and adipose tissue. However, the true starting point may lie in the brain, where disrupted signalling leads to a cascade of events that drive overconsumption, metabolic dysfunction, and ultimately, systemic insulin resistance.
In the previous blog, we looked into the sequence of events leading up to Insulin Resistance in major organs of the body. In this blog, we will explore how brain insulin resistance and impaired gut-brain communication set the stage for the metabolic domino effect.
The Brain's Role in Energy Regulation
The brain is the central regulator of energy balance, continuously monitoring glucose and nutrient availability to adjust hunger, satiety, and energy expenditure.
Two key brain regions play a pivotal role in this:
Hypothalamus: Acts as the command center, responding to insulin and leptin to regulate appetite and metabolism.
Mesolimbic Reward System (Dopaminergic Pathways): Governs food-seeking behaviour, responding to highly palatable foods (rich in sugar and fat) by reinforcing cravings.
Under normal conditions, insulin and leptin suppress hunger and regulate energy intake. However, chronic exposure to ultra-processed foods disrupts this finely tuned system.
Overconsumption of Ultra-Processed Foods and Brain Insulin Resistance
Modern diets rich in sugar, refined carbohydrates, and industrial seed oils cause repeated insulin and glucose spikes. This leads to:
Desensitization of the hypothalamus to insulin and leptin: The brain stops responding to signals that regulate hunger, causing persistent overeating.
Dopamine dysregulation: Highly processed foods overstimulate the reward system, leading to compulsive eating behaviours, similar to drug addiction.
Increased gut-derived inflammation: Processed foods alter gut microbiota, leading to endotoxin leakage into the bloodstream (metabolic endotoxemia), which further impairs brain insulin signalling.
As a result, the brain loses its ability to regulate appetite and metabolism properly, leading to excessive calorie intake and setting the stage for systemic insulin resistance.
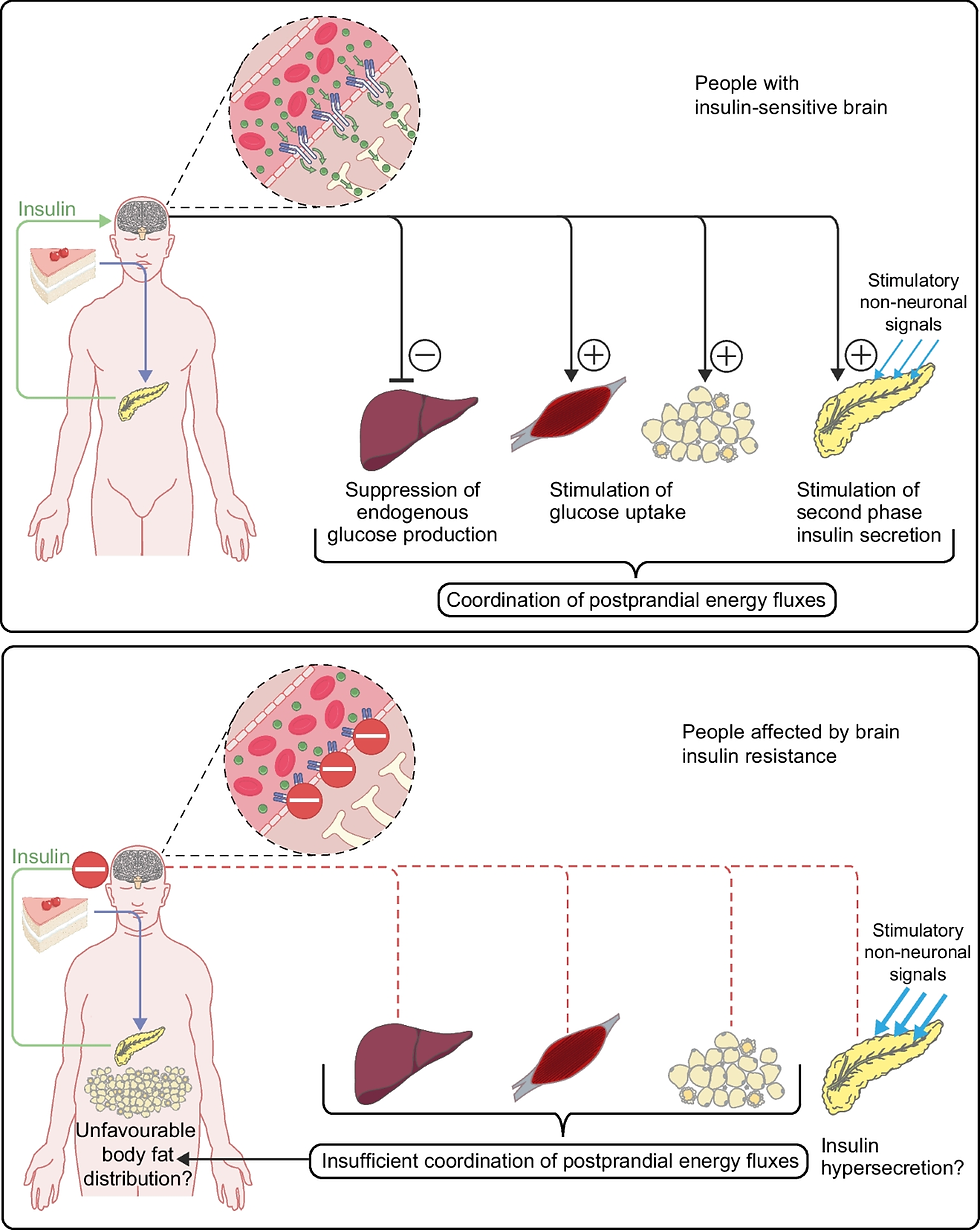
Image Credit: Springer
Brain Insulin Resistance: A Key Factor in Overeating
The brain is traditionally considered insulin-insensitive in most regions, meaning it does not require insulin for glucose uptake. However, insulin does play a role in brain function, particularly in regions that regulate appetite, such as the:
Hypothalamus (controls hunger and satiety).
Prefrontal Cortex (involved in decision-making and impulse control).
Putamen (part of the reward system, linked to hedonic eating).
Studies have shown that in obesity and type 2 diabetes:
The brain becomes resistant to insulin, reducing its ability to regulate appetite and metabolism.
There is gray matter shrinkage in key areas involved in appetite control.
The normal neurocircuitry that signals satiety is distorted, leading to increased food intake and cravings.
How Does the Brain Regulate Appetite?
Under normal conditions, the brain receives hormonal signals from the gut and pancreas during and after a meal, signalling satiety and regulating food intake. Some key hormones involved in this process include:
GLP-1 (Glucagon-Like Peptide-1): Enhances insulin secretion and signals satiety to the brain.
Amylin: Co-secreted with insulin in a 1:1 ratio, amylin helps slow gastric emptying and reduce food intake.
Leptin: Secreted by fat cells, leptin signals long-term energy balance to the brain, preventing excessive food consumption.
However, in obesity and type 2 diabetes, the brain becomes resistant to these hormones, leading to impaired satiety signalling and increased food intake despite already high energy stores.
The Hypoglycemia-Overeating Cycle
Frequent consumption of high-glycemic foods leads to rapid glucose absorption and subsequent insulin spikes. This drives glucose into tissues too quickly, leading to a postprandial drop in blood sugar (reactive hypoglycemia).
This hypoglycemic state triggers:
Increased hunger and cravings for more sugar to rapidly restore glucose levels.
Sympathetic nervous system activation, increasing cortisol and epinephrine, both of which contribute to insulin resistance.
Orexigenic and Anorexigenic Peptides: The Brain’s Role in Appetite Regulation
The brain tightly regulates hunger and satiety through a balance of orexigenic (appetite-stimulating) and anorexigenic (appetite-suppressing) peptides. These neuropeptides are primarily released from the hypothalamus, which acts as the body’s central hub for energy balance.
Orexigenic Peptides (Increase Appetite)
These peptides promote hunger and food-seeking behavior, especially in response to low energy availability.
Key Orexigenic Peptides:
Neuropeptide Y (NPY)
Secreted from the arcuate nucleus (ARC) of the hypothalamus.
Stimulated by fasting, low glucose, and low leptin levels.
Strongly increases appetite and reduces energy expenditure.
Agouti-Related Peptide (AgRP)
Works alongside NPY to enhance hunger.
Inhibits melanocortin receptors (which normally suppress appetite).
Suppressed after food intake.
Ghrelin
Produced by the stomach, but acts on the brain to stimulate NPY and AgRP neurons.
Increases before meals, promoting hunger.
High during fasting and energy deficits.
Anorexigenic Peptides (Suppress Appetite)
These peptides reduce food intake and signal fullness after meals.
Key Anorexigenic Peptides:
Pro-opiomelanocortin (POMC) & α-Melanocyte-Stimulating Hormone (α-MSH)
Produced in the arcuate nucleus and activated by leptin and insulin.
Binds to melanocortin receptors (MC4R) to reduce food intake.
Cocaine- and Amphetamine-Regulated Transcript (CART)
Works alongside POMC to suppress appetite.
Stimulated by leptin and insulin, indicating energy abundance.
Peptide YY (PYY)
Released by intestinal L-cells in response to food intake.
Slows gastric emptying and reduces NPY-stimulated hunger.
Glucagon-Like Peptide-1 (GLP-1)
Secreted from the gut after eating.
Enhances satiety, slows digestion, and stimulates insulin release.
Leptin
Secreted by adipose tissue in proportion to fat stores.
Suppresses hunger by inhibiting NPY/AgRP neurons and activating POMC neurons.
Chronic overeating can lead to leptin resistance, reducing its appetite-suppressing effects.
Insulin
Secreted by the pancreas in response to food intake.
Signals to the hypothalamus to reduce appetite.
Chronically high insulin levels can lead to insulin resistance, impairing satiety signaling.
How This Relates to Insulin Resistance and Appetite Dysregulation
Insulin and Leptin Resistance Disrupt Satiety Signals
Chronically elevated insulin and leptin (from frequent high-carb meals and obesity) cause resistance in the brain.
As a result, the brain fails to recognize energy abundance, leading to persistent hunger and overeating.
Ghrelin Stays Elevated, Driving Hunger
Normally, food intake suppresses ghrelin, but insulin resistance can blunt this response.
This leads to persistent cravings and difficulty controlling meal size.
Dopamine and Reward System Override Satiety Signals
Ultra-processed foods hijack the brain’s reward pathways, leading to excessive dopamine release.
This reinforces compulsive eating, even when energy needs are met.
Gut-Brain Signaling Is Impaired
Poor gut health (due to low fiber intake, inflammation, and dysbiosis) disrupts PYY and GLP-1 signaling, reducing satiety.
This results in frequent hunger and overeating.
Takeaway
The balance between orexigenic and anorexigenic peptides is crucial for healthy appetite regulation. Disruptions—caused by insulin resistance, leptin resistance, and ultra-processed food consumption—can drive chronic hunger, overeating, and fat accumulation, setting the stage for metabolic dysfunction.
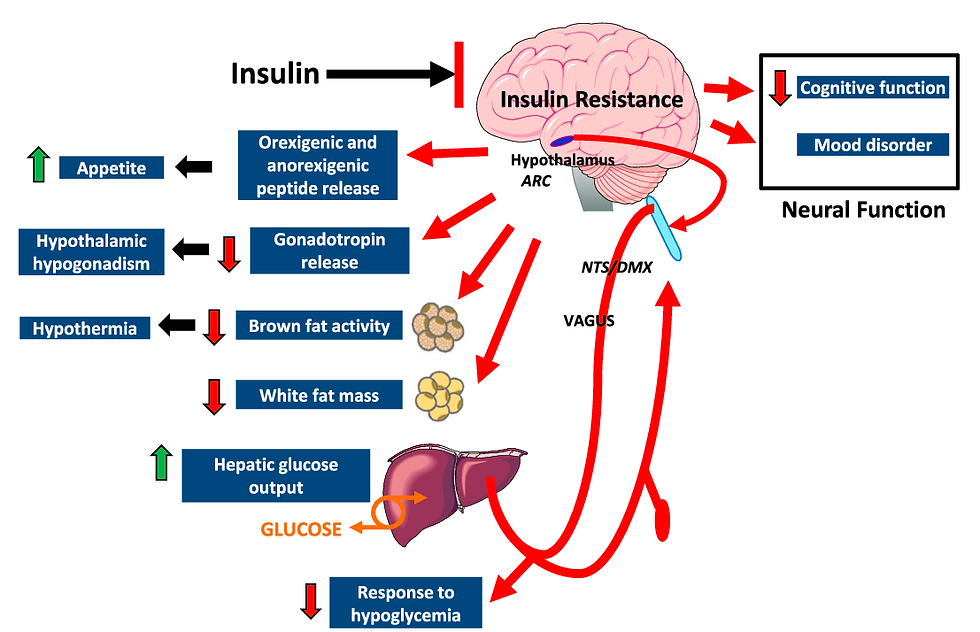
Image Credit: Semantic Scholar
The Link to Cognitive Impairment
Emerging research suggests that brain insulin resistance is not only involved in overeating and obesity but also in neurocognitive dysfunction. Some studies indicate that:
Insulin resistance in the brain may contribute to Alzheimer’s disease, often referred to as “Type 3 diabetes.”
Insulin infusion in individuals with mild cognitive impairment can temporarily improve glucose uptake, suggesting that insulin plays a role in brain function beyond metabolism.
Implications for Treatment
Understanding brain insulin resistance opens up new avenues for treating metabolic disease, including:
GLP-1 receptor agonists (such as semaglutide), which enhance insulin signalling and suppress appetite.
Targeting brain insulin resistance through novel therapeutics that restore proper metabolic signalling.
Lifestyle interventions like exercise, which improve insulin sensitivity not only in the body but also in the brain.
Thus, brain insulin resistance is a critical yet often overlooked aspect of metabolic disease, linking overeating, obesity, and cognitive dysfunction into a unified framework.
The Evolutionary Perspective
The human brain evolved to function optimally even in prolonged states of fasting or food scarcity. Unlike muscles and other tissues, the brain does not rely on insulin for glucose uptake—its glucose transporters (GLUT1 and GLUT3) operate independently of insulin. This was a crucial adaptation for survival, ensuring that even in times of prolonged fasting, the brain received enough energy to function.
As long as blood glucose remains above 50 mg/dL, the brain continues to function normally. This evolutionary adaptation allowed early humans to endure long periods without food, such as during hunting or gathering, without cognitive impairment.
Under normal conditions, a fasting glucose level of ~80 mg/dL is sufficient for brain function.
Even if glucose levels drop to ~50 mg/dL, the brain remains saturated with glucose and functions optimally.
If glucose falls below 40 mg/dL, brain function becomes compromised, leading to confusion, dizziness, and potential unconsciousness.
This mechanism provided a survival advantage. When food was scarce, humans could still think, plan, and hunt effectively, relying on stored body fat and ketones for energy.
Modern Context: The Role of Hyperinsulinemia
In today's world of constant food availability—especially ultra-processed, carbohydrate-heavy foods—this finely tuned system is disrupted. Unlike in ancestral times when blood glucose was tightly regulated, modern diets cause:
Frequent insulin spikes due to high sugar and refined carbohydrate intake.
Reactive hypoglycemia, where excessive insulin secretion leads to a rapid glucose drop, triggering hunger and overeating.
Brain insulin resistance, impairing appetite control and leading to further overconsumption.
Ironically, while the brain is insulin insensitive for glucose uptake, it does respond to insulin for signalling satiety. When insulin resistance develops, the brain stops recognizing insulin’s appetite-suppressing effects, driving further caloric intake and metabolic dysfunction.
This mismatch between our evolutionary adaptations and modern dietary habits sets off the entire cascade of insulin resistance, beginning in the brain and spreading to muscle, adipose tissue, liver, kidneys, and eventually the pancreas.
By understanding this evolutionary context, we can see why reversing insulin resistance requires aligning our modern diet and lifestyle with the metabolic conditions our bodies were designed for.
How to Prevent the First Domino (Brain Insulin Resistance) from Falling
Since brain insulin resistance is the first step in the cascade of metabolic dysfunction, addressing it early can prevent downstream insulin resistance in the muscles, adipose tissue, liver, kidneys, and pancreas. Here’s how to tackle the root cause:
Optimize Nutrient Timing and Meal Composition
Why?Frequent spikes in glucose and insulin lead to dysregulated appetite control and reactive hypoglycemia, driving overeating.
How?
Prioritize protein and healthy fats at meals to slow digestion, enhance satiety, and reduce post-meal insulin spikes.
Limit refined carbohydrates and fructose, especially ultra-processed foods that overwhelm the brain’s satiety signalling.
Incorporate fiber-rich foods (vegetables, nuts, seeds) to slow glucose absorption and prevent rapid blood sugar fluctuations.
Time your meals appropriately—avoid constant snacking and late-night eating, which disrupts insulin signalling.
Regulate Dopamine and Reward System Response
Why?Ultra-processed foods hijack the brain’s dopamine system, reinforcing addictive eating behaviours and making it harder to regulate appetite.
How?
Avoid hyper-palatable foods that contain engineered combinations of sugar, fat, and salt (e.g., chips, pastries, fast food).
Reduce reliance on artificial sweeteners, which may perpetuate cravings and disrupt insulin signalling.
Engage in dopamine-regulating activities such as exercise, meditation, or cold exposure to rebalance the reward system without food dependence.
Prioritize Sleep and Circadian Rhythm Alignment
Why?Sleep deprivation increases insulin resistance in the brain, disrupts hunger hormones (increasing ghrelin and reducing leptin), and alters glucose metabolism.
How?
Aim for 7-9 hours of quality sleep per night.
Maintain a consistent sleep-wake cycle, aligning with natural light exposure.
Avoid screens and artificial light at night, as they disrupt melatonin production and impair metabolic health.
Practice Intermittent Fasting or Time-Restricted Eating
Why?Fasting helps reset insulin sensitivity, lowers brain inflammation, and improves metabolic flexibility.
How?
Start with a 12-hour overnight fast, gradually extending to 14-16 hours if tolerated.
Avoid excessive snacking, allowing insulin levels to return to baseline between meals.
Incorporate occasional longer fasts (24+ hours) if suitable, to promote autophagy and mitochondrial efficiency.
Engage in Regular Physical Activity
Why?Exercise enhances insulin sensitivity in the brain and body, improving glucose metabolism and appetite regulation.
How?
Incorporate daily movement, including walking, strength training, and aerobic exercise.
Exercise in a fasted state occasionally to enhance metabolic flexibility.
Prioritize strength training, as increased muscle mass acts as a glucose sink, improving overall insulin sensitivity.
Manage Stress and Optimize Mental Health
Why?Chronic stress elevates cortisol, which increases glucose levels and promotes brain insulin resistance.
How?
Practice mindfulness, meditation, or deep breathing to lower stress.
Engage in social activities and nature exposure, which support brain health and reduce stress-induced eating.
Limit excessive screen time and doom-scrolling, which overstimulates the brain and contributes to poor sleep and stress.
Support Brain Energy Metabolism with Ketones
Why?The brain functions efficiently on ketones, which can help bypass insulin resistance and improve cognitive function.
How?
Incorporate ketogenic or low-carb meals, allowing the body to produce and utilize ketones.
Use exogenous ketones (if necessary) to supplement brain energy, especially during fasting periods.
Consume medium-chain triglycerides (MCT oil) to provide an alternative fuel source for the brain.
By addressing these key areas, we can prevent brain insulin resistance from taking hold, thereby stopping the entire cascade of metabolic dysfunction before it begins.
Conclusion
The brain is the first domino to fall in the cascade of insulin resistance. By disrupting appetite control and promoting overconsumption, modern diets high in sugar and processed foods initiate a metabolic chain reaction that affects every major organ system.
Addressing brain insulin resistance through diet, exercise, and lifestyle interventions is key to breaking this cycle before it progresses to full-blown metabolic disease.
In the next blog, we will explore skeletal muscle's role in insulin resistance—the body's largest site of glucose disposal and a crucial factor in metabolic health.
*Disclaimer:
The information provided in this blog is for educational and informational purposes only and should not be construed as medical advice. While every effort is made to ensure accuracy, the content is not intended to replace professional medical consultation, diagnosis, or treatment. Always seek the guidance of a qualified healthcare provider with any questions regarding your health, medical conditions, or treatment options.
The author is not responsible for any health consequences that may result from following the information provided. Any lifestyle, dietary, or medical decisions should be made in consultation with a licensed medical professional.
If you have a medical emergency, please contact a healthcare provider or call emergency services immediately.
コメント