In this continuation of Paradigm 3, we delve deeper into the evolutionary connection between our unicellular ancestors and cancer cells. By exploring the role of gene expression in cancer, we examine how key regulatory elements—promoters and enhancers—play a crucial part in orchestrating cellular behaviour. These ancient genetic blueprints (more like a recipe book), which once helped unicellular organisms adapt to their environments, become reactivated under certain conditions in cancer cells, enabling them to thrive and proliferate. This process mirrors the transition from unicellular to multicellular organisms, offering valuable insights into how cancer cells exploit these evolutionary mechanisms to fuel their survival. Through this exploration, we aim to expand our understanding of cancer biology, providing a more comprehensive view of its origins and behaviours.
Chronic Injury and the Evolutionary Mechanism of Cancer
Chronic injury plays a central role in the evolutionary mechanism of cancer. Over time, cells in the body experience continuous damage from environmental factors, such as toxins, radiation, or even internal metabolic processes. When this injury occurs repeatedly and is not severe enough to cause cell death, it creates a type of "selection pressure" on the affected cells. This means that the cells which have developed traits allowing them to survive the damage—such as the ability to resist apoptosis (programmed cell death), proliferate indefinitely, or invade other tissues—are more likely to thrive. Essentially, these cells "adapt" to their damaged environment in a way that grants them a survival advantage, much like the process of natural selection observed in evolutionary biology.
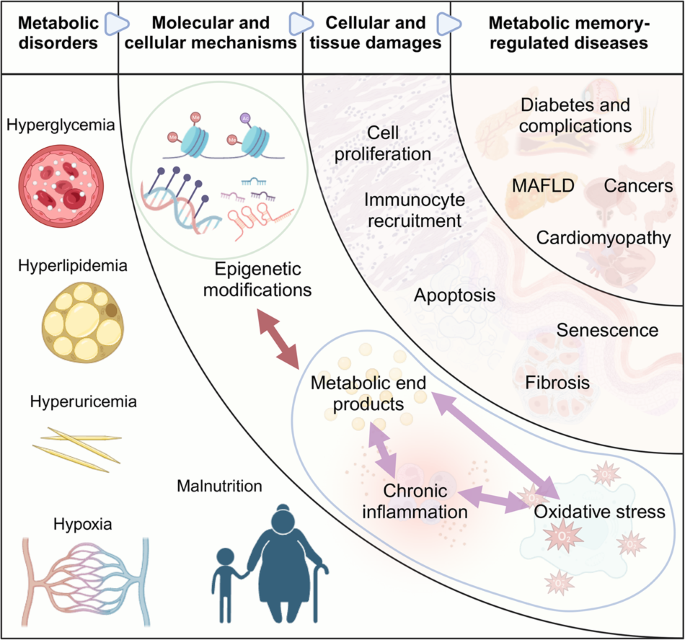
Image Credit: Nature
These survival traits are not random but rather linked to genetic mutations. Interestingly, the mutations associated with cancer often occur in ancient, unicellular genes that predate the evolution of multicellularity. These genes are fundamental to cellular survival and function, and their alterations can lead to the activation of pathways that favour cancerous behaviour. The key to understanding these mutations lies in the concept of phylo-strata, a term that refers to layers of evolutionary genetic history. Research has shown that the mutations found in cancer often cluster within the oldest "strata" of our genes—those inherited from our unicellular ancestors. These ancient genes, which were once critical for the survival of single-celled organisms, continue to play a role in our modern, multicellular bodies. When these genes are re-activated or mutated, they can drive the unchecked growth and survival characteristics seen in cancer cells.
This evolutionary model requires genetic heterogeneity, time, and selection pressure. Chronic injury ensures these conditions, driving cells to revert to primitive behaviours for survival, mirroring cancer’s atavistic nature. All the cells in our body contain the same genetic blueprint—our complete genome, which includes both unicellular-specific and multicellular-specific genes. The difference lies in gene expression, which is tightly regulated and determines which genes are active in a particular cell type at a given time.
As we move forward into discussing gene types and expression, it's important to recognize how these ancient genes are "switched on" in the context of cancer. The regulatory elements of these genes, such as promoters and enhancers, determine when and how these genes are expressed. In a healthy cell, these genes are usually tightly controlled, but in a cancerous cell, they can become dysregulated. Environmental factors, lifestyle choices, and chronic injury contribute to this dysregulation by influencing the expression of these genes. The next section will explore how gene expression and the role of promoters and enhancers are pivotal in orchestrating the cellular behaviours that lead to cancer’s growth and spread, linking back to our evolutionary origins.
1. Gene Types Across Evolution
Unicellular-Specific Genes:
These are ancient genes inherited from our unicellular ancestors.
Found in all cells and typically involved in:
Basic metabolic functions (e.g., glycolysis, DNA replication, protein synthesis).
Cell survival mechanisms.
Stress responses.
They are essential for life and are conserved across nearly all living organisms.
Multicellular-Specific Genes:
These evolved later during the transition to multicellularity.
Found in all cells but selectively activated in multicellular contexts.
Typically involved in:
Cell-cell communication.
Tissue organization.
Differentiation and cooperation between cells.
Apoptosis (programmed cell death).
2. How Cell Types Differ
Every cell in the human body expresses a specific subset of genes depending on:
Cell type and function:
A liver cell will activate genes involved in detoxification (e.g., CYP enzymes) and glucose metabolism, while a neuron will activate genes for neurotransmission.
Both, however, rely on basic "housekeeping" genes (e.g., for energy production) derived from unicellular ancestors.
Developmental stage:
Stem cells activate genes related to pluripotency and division, while differentiated cells activate genes specific to their mature function.
Environment and signals:
Signals from neighboring cells, hormones, and environmental stressors can influence which genes are expressed.
3. Determination of Gene Expression
Gene expression is controlled by several factors:
Epigenetics:
Chemical modifications to DNA (e.g., methylation) and histones determine which parts of the genome are accessible for transcription.
Multicellular-specific genes may be "silenced" in unicellular-like contexts, such as cancer, while unicellular-specific genes may be upregulated.
Transcription factors:
Proteins that bind to DNA and regulate the activation or repression of specific genes.
Different transcription factors are active in different cell types, determining which genes are turned on or off.
Regulatory elements:
Enhancers and promoters dictate when and where genes are expressed.
Microenvironment:
Nutrient availability, oxygen levels, and external stresses influence cellular signaling pathways and gene expression.

Image Credit: thisisepigenetics
Cancer Context
In cancer, the balance between unicellular and multicellular gene expression is disrupted:
Unicellular-Specific Genes Upregulated:
Cancer cells may activate genes linked to survival, growth, and metabolic flexibility—traits aligned with unicellular organisms.
Multicellular-Specific Genes Downregulated:
Genes governing cooperation, apoptosis, and growth regulation may be silenced or mutated, allowing cancer cells to evade normal controls.
This dysregulation drives cancer cells to behave like ancient unicellular organisms, prioritizing their own survival over the organism's health.
The difference in gene expression between unicellular and multicellular genes is similar to how humans, despite all having latent animalistic instincts, express different behaviours depending on the influence of culture, society, and personal experiences. Just as our genes exist in every cell, these animalistic instincts are inherent in every individual. However, the expression of these behaviours is tightly regulated by external factors—social norms, laws, and personal choices—which can guide us to behave in ways that align with societal expectations.
In the same way that society helps channel our behaviour into socially acceptable forms, cells in our body are regulated to express certain genes depending on their function (e.g., cell differentiation or tissue-specific roles). This regulation ensures that multicellular cooperation and order are maintained.
However, when a person is under the influence of alcohol, drugs, or intense anger, these external regulatory factors are diminished. This allows our more primal, "animalistic" instincts to surface. In a similar way, cancer cells lose the regulatory mechanisms that typically control gene expression, allowing ancient, unicellular traits to emerge. These traits—such as uncontrolled growth and survival at the expense of the host—can be thought of as the cancer cell "losing control" over its normal behavior, reverting to a more primal, evolutionary state where its sole focus is survival, similar to how a person under the influence might revert to more base behaviors.
This analogy helps to illustrate how, while our genetic blueprint remains constant, the factors influencing its expression can either suppress or reveal certain traits—whether it's animalistic behavior in humans or cellular functions in cancer.
This comparison emphasizes the balance between control (society for humans or gene regulation for cells) and loss of control (primal instincts for humans or cancerous mutations for cells) in both biological and social contexts.
The Role of Regulatory Elements: Enhancers and Promoters in Gene Expression Control
Regulatory elements, such as enhancers and promoters, play a crucial role in controlling when, where, and how genes are expressed. These elements do not code for proteins themselves but instead regulate the transcription of nearby genes. They are vital in ensuring that the right genes are turned on or off at the right time and in the correct cell type, which is essential for normal development, function, and response to environmental signals.
Here’s a closer look at enhancers and promoters:
Promoters:
Function: A promoter is a DNA sequence located near the beginning of a gene. It serves as a binding site for RNA polymerase and other transcription factors, which are essential for the initiation of transcription—the process that copies the gene’s DNA sequence into messenger RNA (mRNA).
Location: Promoters are typically situated just upstream (before) the gene they regulate.
Role: Promoters control the "on" or "off" status of a gene. When RNA polymerase binds to the promoter, it begins transcribing the gene into mRNA, which is then translated into a protein. The strength of the promoter influences how much mRNA is produced and, ultimately, how much protein is synthesized.
Examples of Transcription Factors: These can include general transcription factors like TFIID and specific factors like activators or repressors, which can enhance or suppress gene expression.
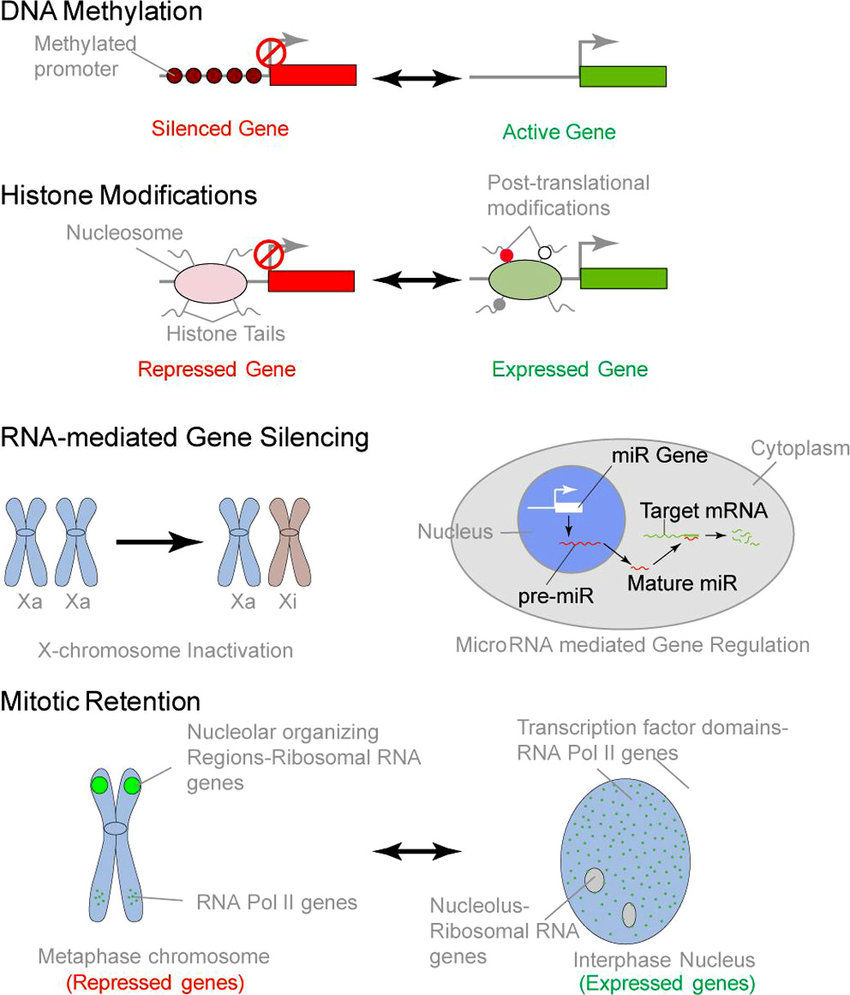
Image Credit: Researchgate
Enhancers:
Function: Enhancers are regulatory sequences that can increase (enhance) the transcription of specific genes, often at significant distances from the gene itself. They are not located directly next to the gene but can be found far upstream or even downstream, and sometimes within introns of the gene.
Location: Enhancers can be located very far from the gene they regulate—sometimes hundreds of thousands of base pairs away—and can function in both directions (upstream or downstream of the gene).
Role: Enhancers boost the activity of promoters, often by providing binding sites for additional transcription factors. These factors help to recruit or stabilize the transcription machinery at the promoter, enhancing the gene’s transcription. In this way, enhancers act like "volume controls" for gene expression.
Cell-Specific Activation: Enhancers can be specific to certain tissues or developmental stages. For example, some enhancers might only be active in brain cells, while others may function only in muscle cells, helping ensure that the appropriate genes are turned on in the appropriate cells.
Cooperation Between Enhancers and Promoters:
Enhancers and promoters often work together to ensure precise gene regulation. While the promoter sets the stage for the transcription machinery to begin its work, enhancers can dramatically increase the level of gene expression.
3D Chromatin Structure: The interaction between enhancers and promoters is often facilitated by the 3D structure of chromatin. Enhancers and promoters may physically interact, even if they are far apart in the linear sequence of DNA. This folding allows enhancers to reach the promoter region and activate transcription, even though they may be separated by large distances on the genome.
Examples in Development and Disease:
Development: In embryonic development, enhancers help regulate genes that control the formation of different tissues. For instance, specific enhancers are responsible for activating genes that form the heart in early development or the eyes in later stages.
Cancer: In cancer cells, mutations in enhancer and promoter regions can lead to the overexpression of oncogenes (genes that drive cancer progression) or the silencing of tumor suppressor genes. Alterations in the normal regulation of these regions can contribute to uncontrolled cell division and cancer.
Here's a table that explains the roles of promoters and enhancers, and how environment, diet, and lifestyle can influence their behavior in the context of gene expression and potentially cancer:
Feature | Promoters | Enhancers |
Definition | DNA sequences that initiate the transcription of a gene. | DNA sequences that increase the transcription of a gene, sometimes from a distance. |
Location | Located directly upstream (before) the gene. | Located at variable distances from the gene—upstream, downstream, or within introns. |
Function | Bind transcription factors and RNA polymerase to start transcription. | Bind transcription factors to boost transcription, even from distant locations. |
Cell Type Specificity | Essential for basic, ubiquitous gene expression across all cell types. | Often tissue-specific; may be active only in certain cell types or under certain conditions. |
Response to Environmental Cues | Regulates how genes respond to environmental signals, such as stress or toxins. | Enhancers can be activated or repressed by environmental factors like hypoxia or inflammation. |
Influence of Diet | Diet can influence the availability of nutrients that support gene expression, which may impact how promoters work (e.g., availability of methyl groups for DNA methylation). | Certain dietary components (like polyphenols) can activate specific enhancers that support healthy cell function. |
Impact of Lifestyle | Physical activity can enhance the efficiency of gene transcription by improving blood circulation and oxygen delivery, impacting promoter activity. | Lifestyle factors like chronic stress or sleep patterns can affect enhancer activity, influencing the expression of genes involved in inflammation or stress response. |
Role in Disease (e.g., Cancer) | Mutations in promoters can lead to overexpression of oncogenes or underexpression of tumor suppressor genes, driving cancer. | Alterations in enhancers (e.g., mutations or environmental influences) can lead to excessive gene activation, including oncogene activation. |
Example in Cancer | Promoter mutations in the MYC gene can result in uncontrolled cell division, a hallmark of cancer. | Overactive enhancers in the MET gene may lead to enhanced cell migration and metastasis in cancer. |
Influence of Stress & Inflammation | Chronic inflammation can activate stress response promoters, increasing the expression of genes involved in inflammation and cell survival. | Stress and inflammation can activate enhancers involved in immune response or cell survival, contributing to cancer cell resistance. |
Impact of Toxins/Carcinogens | Environmental toxins or carcinogens may damage promoters, leading to permanent changes in gene expression and contributing to cancer initiation. | Toxins or carcinogens can modify enhancer regions, leading to the activation of pathways that drive cancer progression. |
Gene Silencing/Activation | Some promoters can be silenced by epigenetic modifications like DNA methylation, reducing gene expression. | Enhancers can also be epigenetically regulated, leading to either enhanced gene activation or silencing depending on the chromatin environment. |
In Summary:
Promoters are the starting points for gene transcription and are crucial for initiating gene expression.
Enhancers amplify gene expression and ensure that genes are activated at the right time and in the right place.
Together, they ensure the proper function of the gene regulatory network, which is essential for maintaining healthy cell function, development, and response to environmental signals.
In the context of cancer, dysregulation of enhancers and promoters can lead to abnormal gene expression, contributing to uncontrolled cell growth, resistance to cell death, and metastasis.
Conclusion: Unraveling the Complex Origins of Cancer
In this blog, we delved into the intricate evolutionary mechanisms that underpin cancer. We explored how cancer cells, much like our ancient unicellular ancestors, rely on survival traits that have evolved over billions of years. Chronic sub-lethal injury acts as a selection pressure, favoring cells with enhanced survival abilities such as immortality, mobility, and uncontrolled growth. As mutations accumulate, they often involve ancient genes tied to unicellular life, leading to the emergence of cancer through processes that mirror the evolutionary shift from single-celled organisms to multicellular complexity.
We also examined the role of gene expression in cancer, with a focus on regulatory elements like promoters and enhancers. These crucial components control the timing, location, and intensity of gene activation, ensuring proper cellular function. When disrupted, these regulatory mechanisms can lead to aberrant gene expression, a hallmark of cancer. The delicate balance of gene regulation is influenced by environmental factors such as diet and lifestyle, which can further complicate the disease’s development.
This discussion sets the stage for the next blog, where we will shift our focus to cancer through a metabolic lens. We will explore how cancer cells rewire their metabolism to thrive, including the shift from oxidative phosphorylation to glycolysis, and the impact of mitochondrial dysfunction on cancer progression. Understanding cancer from this perspective may offer new insights into how we can better target the disease and its underlying processes. Stay tuned as we continue to uncover the complexities of cancer biology, moving beyond traditional genetic theories to more holistic approaches.
Comments